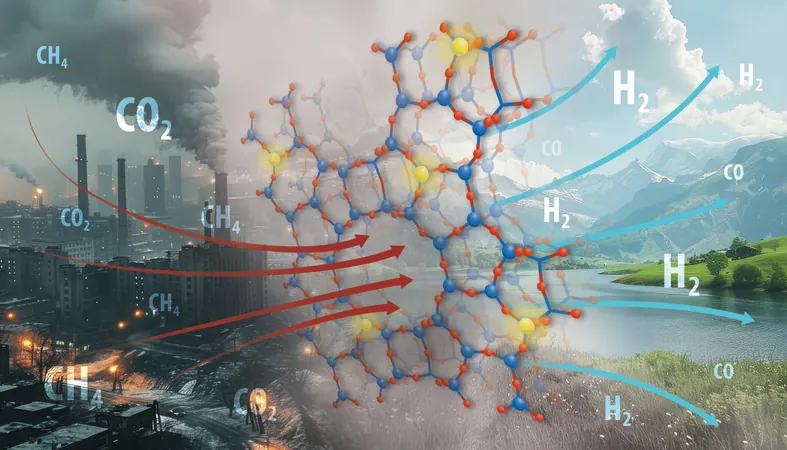
Revolutionary Catalyst Converts Greenhouse Gases into Clean Fuels – Could This Be the Future of Energy?
2024-11-26
Author: Benjamin
Introduction
A groundbreaking chemical reaction has emerged that transforms two notorious greenhouse gases into valuable components for cleaner fuels and feedstocks. Led by a renowned team from the U.S. Department of Energy's Oak Ridge National Laboratory, researchers have discovered a method to prevent catalyst deactivation, a significant hurdle that has plagued this process for decades. This innovative strategy could pave the way for widespread improvements in catalyst technology across various industries.
The Process Behind the Innovation
The research focuses on a process known as dry reforming of methane, where methane and carbon dioxide are converted into syngas—a crucial mixture of hydrogen and carbon monoxide. This syngas is essential for manufacturing a variety of chemicals and fuels globally. "Syngas acts as a precursor for the production of many widely-used chemicals," explained Felipe Polo-Garzon from ORNL, who co-led the study published in Nature Communications alongside Junyan Zhang.
Global Implications of the Research
Enhancing the catalyst used for syngas production holds immense potential for global energy security, especially in regions devoid of oil reserves. Syngas produced from alternative sources like coal or natural gas is vital for creating diesel and gasoline, as well as other chemical products, making this research vital for countries looking to diversify their energy sources.
The Role of Hydrogen and Methanol
Hydrogen generated from syngas can serve as a clean fuel alternative and a vital feedstock for industries producing ammonia for fertilizers. Methanol, another product derived from syngas, plays a critical role in the production of plastics, synthetic materials, and pharmaceuticals, and interestingly, serves as an effective hydrogen carrier.
Challenges in Dry Reforming Technique
Despite its promise, the dry reforming technique has faced considerable challenges due to the high operational temperatures required—over 650 degrees Celsius (1,202 degrees Fahrenheit), which cause the catalyst to deactivate rapidly. Polo-Garzon noted that at these elevated temperatures, catalysts suffer from two critical deactivation processes: sintering, which diminishes available active sites, and coking, where leftover carbon builds up on the catalyst's surface, obstructing chemical reactions.
Comparison with Traditional Methods
Typically, industrial syngas is produced through steam reforming of methane, a process that demands substantial water and energy, while also generating carbon dioxide. Conversely, dry reforming not only eliminates the need for water but actively utilizes the carbon dioxide and methane during the conversion.
Innovative Catalyst Design
The researchers tackled the deactivation issue by meticulously tuning the interactions between metal active sites and the support material during catalyst synthesis, a move that effectively suppressed coke formation and metal sintering. Their innovative catalyst—composed of a zeolite framework with nickel—boasts extraordinary performance in dry reforming with minimal deactivation.
The Importance of Zeolites
Zeolites, akin to sand but with a porous structure that vastly increases surface area, play a crucial role in enhancing catalyst efficacy. The unique structure of the zeolite provides an astounding surface area for reactions; just one gram can offer around 500 square meters of exposed surface.
Advanced Research Techniques
This high-performance catalyst was synthesized at ORNL's Center for Nanophase Materials Sciences. Through a series of advanced studies, including infrared spectroscopy and X-ray absorption spectroscopy, the team delved deep into the bonding structures of nickel within the catalyst, leading to a better understanding of how to fortify the catalyst against degradation under intense conditions.
Future Directions
Next steps involve developing new catalyst formulations that can withstand a broader scope of operating conditions. "Our goal is to find alternative methodologies for activating reactant molecules, thereby surpassing thermodynamic limitations," Polo-Garzon revealed.
Conclusion
By employing rational design principles rather than trial and error, the team aims to create a spectrum of catalysts that can be utilized in various industrial processes, ultimately paving the road for a more sustainable energy future.
Implications for Climate Change
Could this revolutionary achievement signal a new era in combating climate change? The ability to transform detrimental greenhouse gases into useful industrial materials not only addresses pressing environmental challenges but also reshapes the landscape of energy production and chemical manufacturing. As further developments unfold, the implications of this research could alter the way industries approach emissions and energy sourcing—propelling us closer to a cleaner, greener planet.