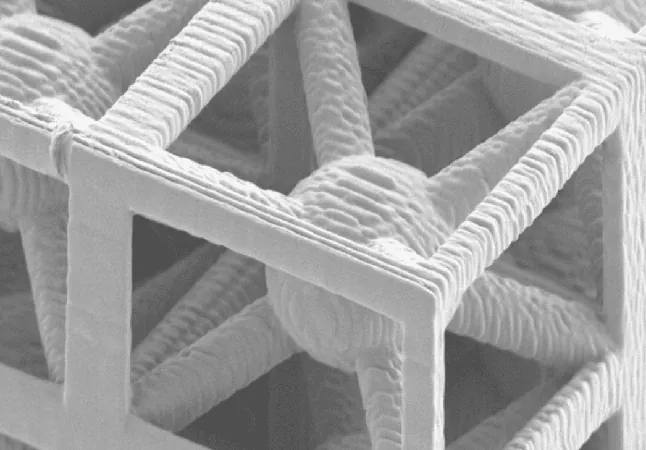
Breakthrough in Tunable Ultrasound Propagation with Microscale Metamaterials
2024-12-15
Author: Rajesh
Introduction
In an exciting advancement in the field of acoustic metamaterials, researchers from MIT have unveiled a groundbreaking design framework that enables precise control over ultrasound wave propagation at the microscale. This innovative research has significant implications for applications ranging from medical diagnostics to mechanical computing.
Overview of Acoustic Metamaterials
Acoustic metamaterials—engineered materials with custom geometries designed to manipulate the behavior of sound waves—have traditionally faced challenges due to their large size and limited frequency capabilities. Carlos Portela, the Robert N. Noyce Career Development Chair and a mechanical engineering assistant professor at MIT, points out the immense potential of these materials: "Their lightweight and strong characteristics, combined with tunable acoustic properties, make them ideal for extreme-condition engineering applications."
Research Collaboration and Publication
The collaborative study, coauthored by Portela along with researchers Rachel Sun, Jet Lem, Yun Kai from the MIT Department of Mechanical Engineering, and Washington DeLima from the U.S. Department of Energy's Kansas City National Security Campus, recently published their findings in the journal *Science Advances*. The paper titled “Tailored Ultrasound Propagation in Microscale Metamaterials via Inertia Design” details their innovative approach of strategically positioning microscopic spheres within a metamaterial lattice. This configuration allows for the tuning of ultrasound waves' travel characteristics, effectively facilitating wave guiding and focusing capabilities.
Experimental Methodology
The team's use of nondestructive, high-throughput laser-ultrasonics enabled them to experimentally manipulate elastic-wave velocities within the microscale structures. Their research demonstrated an acoustic demultiplexer, a device capable of splitting an acoustic signal into various output signals—an important development for technologies reliant on precise signal processing.
Insights and Future Directions
"What we discovered is that simple geometric adjustments can significantly expand the tunable properties of these metamaterials," Portela explains. This capability paves the way for the design and creation of advanced microscale devices, potentially revolutionizing areas such as ultrasound imaging and communication through sound waves.
Mechanical Principles of Wave Behavior
Moreover, the study emphasizes the mechanical principles underpinning ultrasound wave behavior in metamaterials. The research articulates how altering geometric features—through minor modifications in mass and stiffness—can yield pronounced changes in the material's dynamic properties.
Research Impact and Future Outlook
Sun, the lead author of the study, remarked on the transformative nature of their findings: "The elegance of this framework lies in its ability to connect physical material attributes with geometric designs. By manipulating mass on a spring-like lattice, we can observe fascinating phenomena regarding stiffness and wave velocity." As the research continues to unfold, it stands to provoke further exploration in the fabrication of microscale acoustic metamaterials, opening doors to revolutionary medical and technological applications. As scientists hone in on these fabrications, the potential for enhanced devices that can adapt to complex environments seems limitless. Stay tuned—this innovation could change the landscape of ultrasound technology forever!