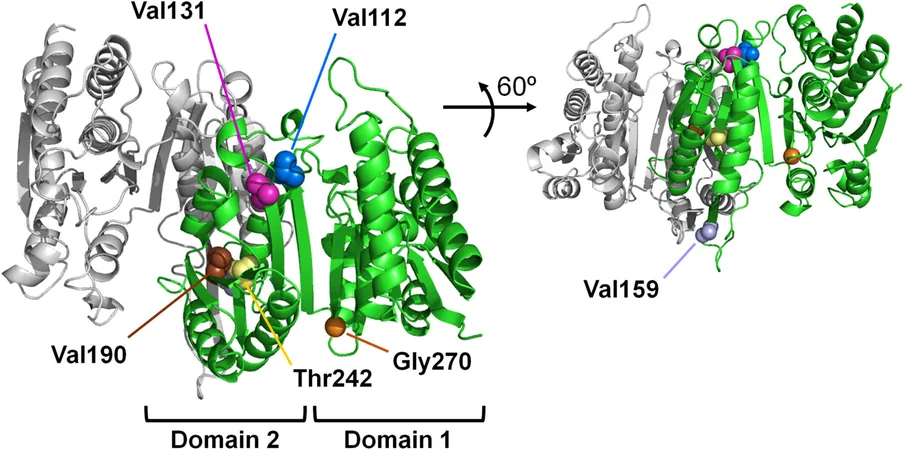
Groundbreaking Discoveries Reveal How Enzymes Adapted to Survive in Cold Climates!
2025-03-25
Author: Ming
Introduction
In an extraordinary exploration of life’s adaptation over billions of years, scientists have made groundbreaking discoveries about enzymes—critical proteins that catalyze biochemical reactions in living cells. These enzymes have evolved to thrive in various temperature ranges, showcasing nature's incredible ability to adapt.
Enzymes and Temperature
For most organisms, including humans, enzymes operate best at around body temperature (37 °C). Deviating from this optimal range can drastically hinder enzyme performance. However, some unique organisms, known as extremophiles, can flourish in the most extreme environments, including boiling hot springs or the freezing waters of the polar regions. These adaptations have resulted in enzymes that are uniquely suited for their harsh habitats.
Adaptations of Extremophiles
Enzymes from thermophiles, for example, display resistance to high temperatures and maintain their catalytic efficiency in those conditions, but their performance drops significantly as temperatures cool. Conversely, enzymes from psychrophiles (cold-loving organisms) are optimized for low temperatures and excel when temperatures plummet. A fascinating theory suggests that the first living organisms were thermophiles, gradually adapting as the Earth experienced cooling over millennia.
Molecular Structure and Evolution
The flexibility of an enzyme’s molecular structure plays a crucial role in its ability to function at lower temperatures. Yet, the intricate molecular mechanisms behind this evolutionary adaptation remain largely misunderstood. Insights into how these enzymes evolved can not only unravel mysteries of evolutionary biology but can also pave the way for bioengineering efforts aimed at designing enzymes suited for varying temperature environments.
Ancestral Sequence Reconstruction (ASR)
Given that the ancestral enzymes of such adaptations no longer exist, scientists have turned to a technique known as ancestral sequence reconstruction (ASR). This method blends molecular phylogenetics with genetic engineering to reconstruct the sequences of lost enzymes based on closely related species that are still alive today.
The Case Study of 3-Isopropylmalate Dehydrogenase (IPMDH)
One particular enzyme, 3-Isopropylmalate dehydrogenase (IPMDH)—crucial for synthesizing leucine, one of the 20 amino acids that form proteins—serves as a prime focus for investigating adaptations linked to cold temperatures. A research team led by Professor Satoshi Akanuma from Waseda University, along with other esteemed researchers, analyzed the evolutionary trajectory of IPMDH, tracing its lineage from an ancient thermophile ancestor to modern-day Escherichia coli.
Research Findings
Their findings, published in the journal Protein Science, reveal that the efficiency of IPMDH at lower temperatures saw a remarkable jump, particularly between two intermediate ancestral forms, Anc05 and Anc06. This transition raised questions: What sparked such a significant increase in enzymatic performance?
Molecular Dynamics and Structural Transition
By comparing the amino acid sequences of these ancestral enzymes, the scientists employed a technique known as site-directed mutagenesis to pinpoint specific mutations that enhanced enzyme activity at 25 °C. Astonishingly, they discovered that three pivotal mutations occurred further away from the active site than previously thought, challenging conventional wisdom that temperature adaptations mainly stem from changes at active sites.
Through molecular dynamics simulations, the researchers identified a significant structural transition between Anc05 and Anc06. This shift allowed Anc06 to adopt a partially closed conformation that efficiently reduced activation energy, thereby enhancing enzymatic performance in colder temperatures.
Historical Context and Climate Impact
This pivotal evolution occurred approximately 2.5 to 2.1 billion years ago during the Great Oxidation Event, which marked a dramatic transformation in Earth’s atmosphere and an overall cooling trend. The researchers theorize that this climate shift could have spurred the adaptation of various enzymes to function more effectively at lower temperatures.
Implications for Bioengineering
The implications of this research extend beyond academic interest; the knowledge gained could revolutionize the bioengineering of enzymes for use in biotechnology, pharmaceuticals, and environmental applications. As Professor Akanuma states, "By applying our findings to other enzymes, we can further understand how life has ingeniously adapted to the Earth's fluctuating environments over billions of years."
Conclusion
Stay tuned as we uncover more about these awe-inspiring adaptations and their role in the future of science and technology!