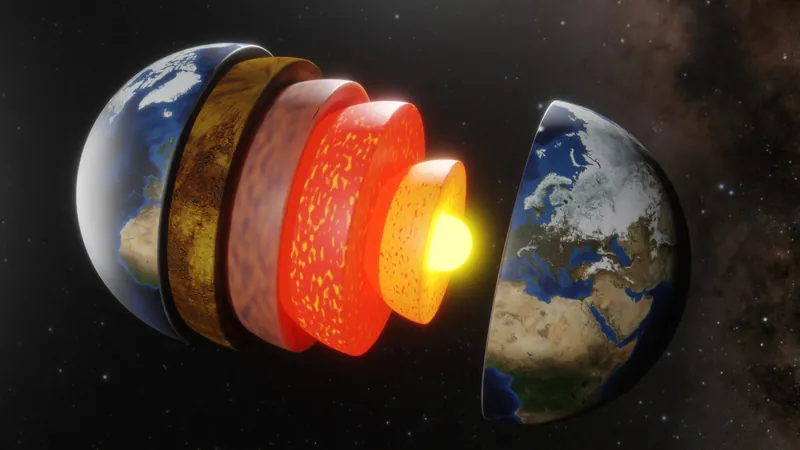
Groundbreaking Simulation Reveals Secrets of Earth's Magnetic Field and Paves the Way for Advanced AI Technology
2024-12-16
Author: Li
Understanding the Earth's Magnetic Field
How does our Earth generate its magnetic field? While we have a grasp on the fundamentals, many intricate details have remained shrouded in mystery. A pioneering team of researchers from the Center for Advanced Systems Understanding at the Helmholtz-Zentrum Dresden-Rossendorf, Sandia National Laboratories in the U.S., and the French Alternative Energies and Atomic Energy Commission is turning the tide with an innovative simulation method that could revolutionize our understanding of the Earth’s core.
The researchers presented this groundbreaking method in the prestigious Proceedings of the National Academy of Sciences. This simulation not only models atomic behavior but also maps out the magnetic properties of materials, representing a significant leap forward in both geophysics and the realm of neuromorphic computing—an approach spearheading more efficient artificial intelligence (AI) systems.
The Earth's magnetic field is a vital protective barrier against cosmic radiation and solar winds, generated by the geodynamo effect, which is complex and not yet fully understood. Attila Cangi, the Head of the Machine Learning for Materials Design department at CASUS, explains, "The Earth's core is predominantly iron. As we descend into the core, both temperature and pressure increase, influencing whether the material is solid or molten."
The outer core remains in a fluid state whereas the inner core is solid. The interplay of these conditions creates an environment where electrically charged, liquid iron flows around the solid inner core, driven by the Earth’s rotation. It is this movement that generates electric currents, and consequently, the magnetic field we rely on.
However, several unanswered questions plague scientists: What is the precise structure of the Earth's core? What roles do additional elements, speculated to exist alongside iron, play? The answers could significantly impact our understanding of the geodynamo effect.
Seismic wave experiments provide some hints, with scientists sending shockwaves through the Earth and measuring their rebounds with advanced sensors. According to Svetoslav Nikolov, a lead author from Sandia National Laboratories, "Our measurements suggest that beyond iron, the core's composition is far more complex than current simulations assume."
Revolutionary Simulation Technique
Harnessing cutting-edge technology, the research team has made substantial progress by developing a novel simulation approach called molecular-spin dynamics. This method ingeniously melds two distinct simulation techniques: molecular dynamics, which focuses on atomic movement, and spin dynamics, which addresses magnetic properties.
Julien Tranchida, a physicist with the CEA, underscores the method's significance: "By combining these two approaches, we can analyze the interplay of magnetism under extreme high-pressure and high-temperature environments with unprecedented detail."
The team simulated the behavior of 2 million iron atoms, along with their spins, to scrutinize how mechanical and magnetic properties intertwine. They leveraged artificial intelligence to hone the force fields—fundamental interactions between atoms—leading to highly precise modeling. This necessitated the use of powerful computational resources due to the complexity involved.
By applying conditions analogous to those within the Earth’s core, including pressure waves that simulate heating and compression, the team discovered critical behaviors of iron under various shock wave speeds. At slower speeds, iron retained a solid state, showcasing diverse crystal forms, while at higher velocities, it melted into a primarily liquid state. Significantly, they observed that magnetic properties notably influenced these core behaviors.
Mitchell Wood, a materials scientist at Sandia National Laboratories, stated, "Our simulations align closely with the experimental data and suggest that under specific conditions, a little-known phase of iron, the BCC phase, could stabilize and potentially impact the geodynamo process."
Implications for AI and Materials Science
This simulation technique extends beyond simply illuminating geological mysteries; it also has the potential to catalyze breakthroughs in technological fields, particularly in materials science. Cangi's team envisions utilizing this method to advance neuromorphic computing devices, inspired by the human brain's architecture. This new class of hardware could revolutionize AI processing, making it not only faster but also much more energy-efficient.
Additionally, this research opens up avenues in data storage technology. Magnetic domains along miniature nanowires could function as ultra-fast and energy-efficient storage media—tantalizing prospects as society's demand for data processing accelerates.
Cangi concludes with optimism, “Currently, no accurate simulation methods exist for these technologies. However, our innovative approach can realistically model the necessary physical processes, significantly expediting the development of IT innovations.”
As researchers continue to unveil the complex tapestry of our Earth’s core—while simultaneously pushing the boundaries of AI technology—it becomes evident that the intersection of geophysics and computing is set to redefine our understanding of both the planet we inhabit and the technological future we are crafting. Don't miss out on the upcoming revelations that could change everything we know!