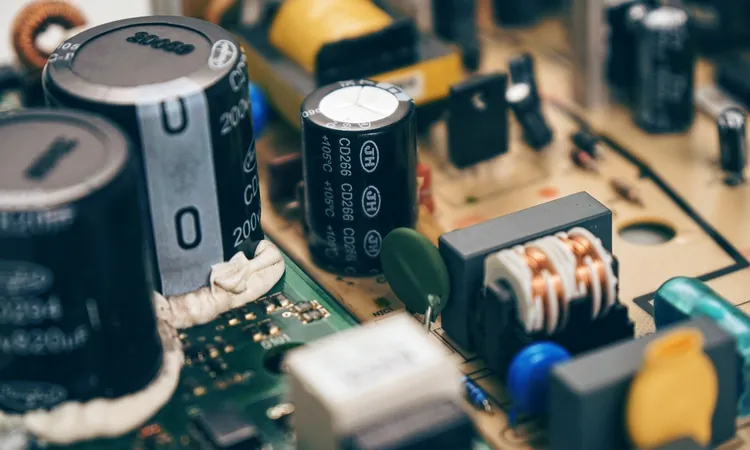
Revolutionary New Model of Electric Double Layer Could Transform Energy Storage!
2024-10-01
Introduction
A groundbreaking new model has been developed that is set to redefine our understanding of how ion-electrode interactions occur, shedding light on the ability of devices to store electric charge efficiently. Fittingly published in ChemPhysChem, this study showcases theoretical predictions that align closely with experimental data, paving the way for more advanced and efficient supercapacitors essential for the future of portable electronics and electric vehicles.
Batteries vs Supercapacitors
In the world of energy storage, batteries remain the most recognized option, capable of delivering a consistent energy stream until depleted. However, supercapacitors stand out for their unique ability to discharge energy in swift bursts, providing power on demand. Imagine a battery as a slow-draining reservoir, while a supercapacitor is akin to a high-octane water balloon—filled quickly and released explosively. This rapid discharge capability is vital for applications requiring immediate power, such as in hybrid cars and emergency systems.
Efficiency Challenges
The efficiency of a supercapacitor hinges on its internal resistance—typically higher than that of batteries—which allows it to handle significant currents. The accumulation of energy within a supercapacitor is made possible through the electric double layer (EDL), a phenomenon where charge separation occurs at the interface of the electrode and the electrolyte.
Key Parameters of Supercapacitors
Key parameters for any capacitor's charge storage capability include the surface area of its plates, their separation distance, and the dielectric material utilized. Given that the electrolyte layer in supercapacitors is only a few nanometers thick, combined with the vast surface area provided by porous electrodes, supercapacitors can store energy in volumes that far surpass traditional capacitors.
Research Insights
Delving deeper, this research sheds light on how electrochemical processes at the quantum level influence the behavior of the electric double layer, emphasizing the need for detailed studies on EDL properties to enhance electrical devices' performance.
Team and Methodology
Led by researchers from HSE MIEM and the Semenov Research Centre of Chemical Physics, the team utilized a modified Poisson-Boltzmann equation to create their model. This novel approach factors in a variety of interactions—between ions and water molecules, the effects of electric fields, and the spatial constraints at the electrode surface. Such intricate considerations allowed them to accurately depict the differential electrical capacitance profiles, crucial for understanding charge accumulation as voltage fluctuates.
Study of Ion Interactions
In their study, the team examined aqueous solutions like sodium perchlorate (NaClO4) and potassium hexafluorophosphate (KPF6) at the interface with a silver electrode. The findings not only confirmed the model's predictive power concerning the electric double layer's structure but also highlighted its adaptability for complex electrochemical systems, including mixtures of different electrolytes.
Expert Commentary
Yury Budkov, Leading Research Fellow at the Laboratory for Computational Physics at MIEM HSE, emphasizes the importance of their work: “Our theoretical predictions align seamlessly with experimental data. This is vital since gauging differential electrical capacitance during experiments is often intricate and labor-intensive.” The unveiled model could provide crucial insights where empirical data is challenging to obtain.
Future Directions
This research marks the beginning of a series aimed at establishing a robust theory of the electric double layer at metal-electrolyte interfaces, with plans to broaden the model to account for stronger ion-electrode interactions typically found in numerous practical applications.
Conclusion
Budkov remarks on the significance of this advancement: “The enhanced model aims to incorporate additional elements that affect the performance of modern electrochemical devices. This is critical as we look to innovate supercapacitors for diverse applications, ranging from handheld gadgets to the latest electric vehicle technologies.”
With this innovative model, the future of electric energy storage looks brighter than ever—are we on the brink of the next generation in supercapacitor technology? Stay tuned!