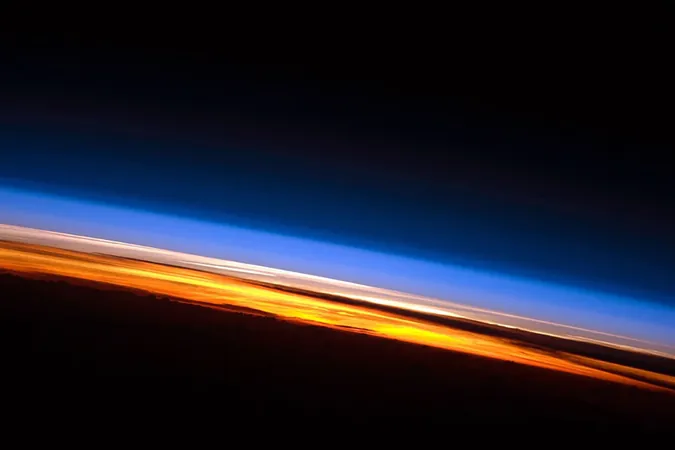
Revolutionary Statistical Method Unveils Secrets of Early Earth and Exoplanet Atmospheres!
2024-11-18
Author: Mei
In a groundbreaking study, researchers from Penn State have introduced a revolutionary statistical method that could transform our understanding of early Earth's atmosphere and assist in the quest for habitable planets beyond our solar system. As solar energy reaches our planet, some of this radiation triggers vital chemical reactions, including the formation of ozone and the breakdown of gas molecules. This new approach promises to enhance existing models of these complex processes.
Published in the journal *JGR Atmospheres*, the team's research utilizes a statistical technique known as correlated-k, which can refine current photochemical models used to investigate atmospheric conditions on early Earth. This methodological shift holds immense potential for interpreting the atmospheric composition of other planets as new observatories come into operation over the next few decades, providing crucial data for exoplanet studies.
"Detecting potential life on other planets hinges on identifying biosignatures," explains Jim Kasting, co-author of the study and Evan Pugh University Professor Emeritus of Geosciences at Penn State. "By using advanced telescopes to analyze atmospheric spectra, our photochemical model can calculate chemical conditions in these atmospheres, significantly aiding the search for extraterrestrial life."
The prevailing models for atmospheric calculations of both early Earth and Earth-like exoplanets predominantly rely on a one-dimensional (1D) photochemical model originally developed at Penn State. According to Aoshuang Ji, who earned a Ph.D. in geosciences from the university and conducted this pivotal research, the introduction of the correlated-k method to the existing model represents a vital step in enhancing its accuracy and applicability.
The role of ultraviolet radiation from the sun is crucial for ozone formation in our atmosphere. Oxygen molecules absorb this radiation, resulting in the fragmentation into free oxygen atoms, which can then recombine to form ozone. This essential process also prevents other atmospheric gases from breaking down due to excessive radiation exposure. However, modeling these complex interactions—particularly at the specific Schumann-Runge (SR) bands—is notoriously challenging.
While current models like the Whole Atmosphere Community Climate Model excel in simulating today's atmosphere, they often overlook crucial factors such as scattering—which is how particles in the atmosphere redirect solar radiation. This is especially significant in low-oxygen environments, like that of early Earth. The Penn State 1D model, despite its strengths, has relied on outdated band models and does not accurately account for temperature-dependent chemical reactions at low oxygen levels.
The research team’s transition to the correlated-k approach has paved the way for improved accuracy across various oxygen levels and temperature conditions. Ji noted, "This method simplifies the problem by organizing wavelengths into bands, under the assumption that absorption properties are correlated in a predictable manner. It strikes a balance between computational efficiency and accuracy, enabling better models of atmospheric absorption and scattering."
This innovative approach opens new avenues for future research on the atmospheres of early Earth and habitable exoplanets. It highlights the potential of correlated-k to revolutionize our understanding of planetary atmospheres, making the search for life beyond our planet more promising than ever. As scientists embark on this exciting journey, who knows what other mysteries of the cosmos we might soon unravel?