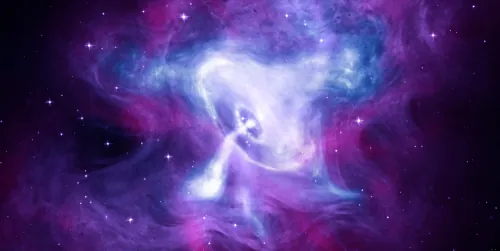
Unlocking the Mysteries of Neutron Stars: The Role of Computer Simulations
2025-01-06
Author: Arjun
Unlocking the Mysteries of Neutron Stars: The Role of Computer Simulations
The enigmatic world of neutron stars is being unraveled through cutting-edge computer simulations, offering groundbreaking insights into their internal properties, including pressure and mass limits.
Despite being among the densest celestial bodies in the universe, studying neutron stars is a formidable challenge. Located approximately 400 light-years away from Earth, sending a probe would require a staggering half a million years with current technology. Through telescopes, neutron stars appear merely as distant points in the cosmos, due to their small size—comparable to that of a city. Furthermore, no earthly laboratory can replicate the extreme conditions within these stellar remnants, where densities exceed those found in atomic nuclei.
Scientists have sought to comprehend these objects using complex theoretical frameworks combined with advanced computer simulations. A team led by Ryan Abbott from the Massachusetts Institute of Technology (MIT) has emerged as pioneers in this niche, providing new, robust constraints regarding neutron stars' interiors. Their findings suggest a higher potential for neutron stars to achieve masses exceeding previous limits, as indicated by a relatively high estimate of sound speed within their cores.
Neutron stars are significantly influenced by quantum chromodynamics (QCD), which describes the fundamental interactions of quarks and gluons within protons and neutrons. While the principles are theoretically established, accurately solving QCD for neutron stars presents a unique challenge. Standard computational strategies struggle due to the extreme density of neutron star matter, where perturbation theory—which relies on small parameter expansions—breaks down. Nonetheless, researchers have developed alternative strategies like lattice QCD, which discretizes the space-time fabric to tackle these challenges.
In an innovative approach, Abbott's team employed the concept of isospin—a form of nuclear charge that differentiates between protons and neutrons—to explore states of matter where protons significantly outnumber neutrons. Previous studies indicated that nuclear matter pressure is inherently constrained, allowing Abbott's group to methodically investigate high-density scenarios. Their collaboration ran extensive numerical simulations on top-tier supercomputers to extrapolate data beyond the limitations of lattice QCD, providing unprecedented insights into high-energy states of nuclear matter.
One of the monumental discoveries from this research is the identification of a superconducting phase in high isospin density nuclear matter, characterized by the superconducting gap parameter, which informs the stability and energy efficiency of material under extreme conditions. The calculated pressure contrasts with that of nonsuperconducting matter, yielding more precise figures than prior analytical approaches.
Furthermore, the researchers uncovered that the speed of sound within this exotic nuclear matter transcends the established conformal bound—a key limit related to the theoretical maximum mass of neutron stars. This boundary is crucial: it implies that neutron stars could potentially exceed the previously accepted upper mass limit of approximately two solar masses without collapsing into black holes, thereby reshaping our understanding of their stellar evolution.
The implications of these rigorous results extend beyond the current research. With precise constraints on isospin nuclear matter, astrophysicists now possess a valuable reference point for validating modeling approaches related to neutron stars. New computational techniques using lattice QCD are being proposed, opening avenues for deeper explorations of these extraordinary objects.
As researchers continue to refine their models, there are prospects for gaining further insights into properties of neutron stars, such as viscosity and thermal conductivity, which are essential for deciphering their cooling mechanisms and spin-down processes. The future of computational astrophysics regarding neutron stars looks exceptionally promising, heralding a new era of discovery and understanding in the field.