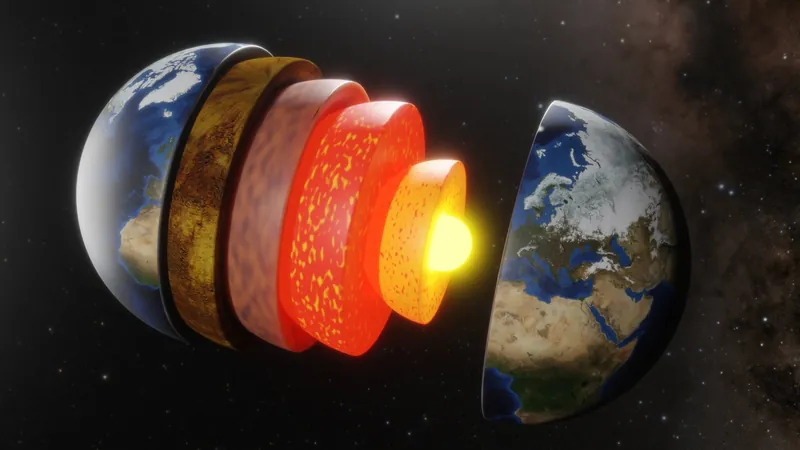
Revolutionary Simulation Technique Uncovers Secrets of Earth's Magnetic Field and Paves the Way for Advanced AI Technology
2024-12-16
Author: Michael
How does Earth generate its magnetic field? While scientists have made significant strides in understanding this phenomenon, many intricacies remain elusive. A groundbreaking team of researchers from the Center for Advanced Systems Understanding (CASUS) at Helmholtz-Zentrum Dresden-Rossendorf, Sandia National Laboratories in the U.S., and the French Alternative Energies and Atomic Energy Commission, has unveiled a novel simulation method promising to illuminate the mysteries of the Earth's core.
Published in the Proceedings of the National Academy of Sciences, this innovative approach simulates atomic behavior alongside the magnetic properties of materials, bridging gaps in geophysical research and holding implications for the evolution of neuromorphic computing—an exciting frontier in efficient AI systems.
The significance of Earth's magnetic field cannot be overstated; it acts as a shield against cosmic radiation and solar winds, integral for maintaining life. This vital magnetic field is produced by the geodynamo effect, which occurs in the liquid outer core—mainly composed of iron. According to Attila Cangi, head of the Machine Learning for Materials Design department at CASUS, "As you approach the Earth’s core, temperature and pressure escalate, causing materials to transition states between solid and liquid."
The flow of electrically charged liquid iron around the solid inner core, fueled by Earth's rotation and convection currents, generates electric currents essential for creating the planet's magnetic field. Despite established theories, many questions linger: what exactly is the structure of the Earth's core, and what roles do the additional elements alongside iron play in this process? Understanding these factors could significantly alter our comprehension of the geodynamo effect.
Recent experiments involving seismic waves traversing the Earth reveal new clues. Svetoslav Nikolov from Sandia National Laboratories notes, "Our measurements indicate that the core isn't merely composed of iron." Current simulations based on an iron-only model fail to align with what scientists have observed.
A Breakthrough in Simulation Techniques
The research team made remarkable advancements by developing an innovative simulation method, known as molecular-spin dynamics. This technique effectively merges two separate simulation frameworks: molecular dynamics, focused on atomic motions, and spin dynamics, which considers magnetic properties.
Julien Tranchida, a physicist at CEA, stresses the importance of this integration, "With our combined methods, we explored the effects of magnetism under extreme temperature and pressure conditions previously deemed unattainable." By simulating the behavior of 2 million iron atoms and their magnetic spins, the team could analyze the interactions between mechanical and magnetic properties under various conditions.
Artificial intelligence enhances this process by employing machine learning to determine high-precision force fields—key interactions between atoms—requiring cutting-edge computing resources.
Once set, the digital model endured the same temperature and pressure that exist within Earth's core. Results revealed that at lower speeds of shock waves, iron remained solid, while faster waves resulted in a predominantly liquid state. Crucially, they found that magnetic influences alter material properties significantly.
Mitchell Wood, a materials scientist at Sandia National Laboratories, asserts, "Our simulations align well with experimental findings, suggesting that under specific conditions, a unique phase of iron could stabilize and influence the geodynamo." This hypothesized phase, referred to as the BCC phase, has yet to be observed experimentally but may shed light on essential geophysical mysteries if validated.
Fueling Next-Generation AI and Tech Innovations
In addition to unveiling Earth's inner workings, this simulation method can spearhead advancements in materials science. Cangi envisions applying this technique to create neuromorphic computing devices—hardware inspired by human brain processes that may eventually outperform conventional AI algorithms regarding speed and energy efficiency.
Furthermore, storing data using magnetic domains along minuscule nanowires presents another promising research area, offering quicker and more efficient storage than current technologies. Cangi insists, "No accurate simulation methods exist for these applications, but I am optimistic that our innovative approach can enhance the modeling of these critical physical processes, fast-tracking the technological evolution of IT solutions."
With the dual potential of deepening our understanding of Earth's core and revolutionizing AI hardware, this research represents a monumental leap forward. The possibilities are staggering—could we be on the brink of groundbreaking technological innovation? Stay tuned!