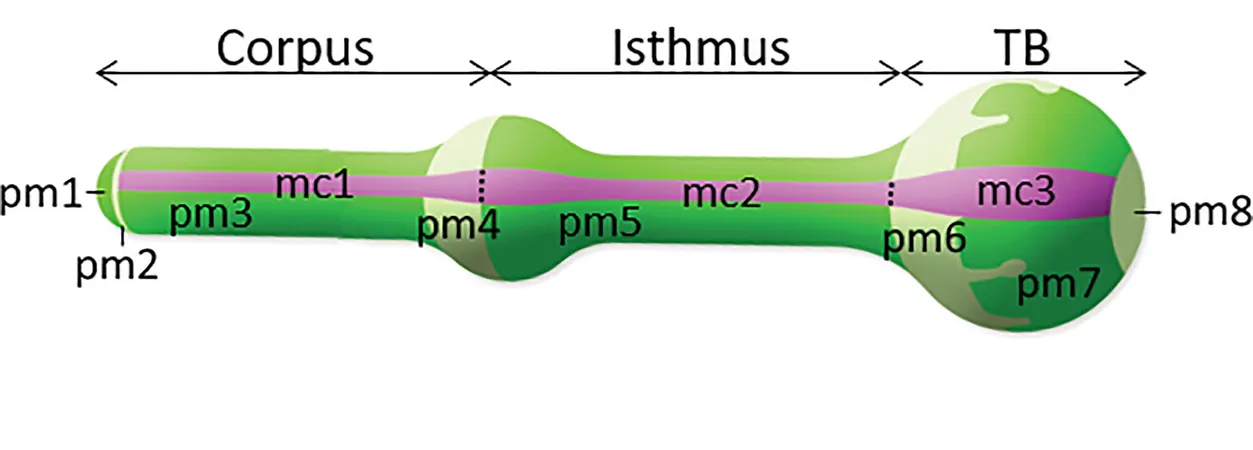
Unveiling the Secrets of Life: How a Tiny Worm is Changing Our Understanding of Biology
2024-11-18
Author: Ming
Unveiling the Secrets of Life: How a Tiny Worm is Changing Our Understanding of Biology
The seemingly simple throat of the roundworm *Caenorhabditis elegans* is turning out to be a goldmine for scientific discovery, showcasing the incredible complexity of life’s physiological processes. Recent research from the prestigious Weizmann Institute of Science highlights how these tiny nematodes, which have become beloved subjects in the world of biological sciences, can offer profound insights into the mechanisms that govern living organisms.
This groundbreaking study led by Dr. Dana Sherman and Professor David Harel demonstrates the power of advanced mathematical and algorithmic models in producing a fine-grained understanding of the biological systems at work within these tiny creatures. The researchers concentrated on the nematode's pharynx—the organ responsible for feeding—building a sophisticated model to simulate and analyze the intricate swallowing motions that allow the worm to ingest food.
Why C. elegans? The Ultimate Biological Model
*C. elegans* has earned its reputation as a model organism through decades of research and discoveries, contributing significantly to the fields of genetics, neurobiology, and developmental biology. With around just 1,000 cells, its transparent body allows scientists to observe biological processes in real-time. This tiny nematode was the first multicellular organism to have its genome completely sequenced, providing a foundational resource for genetic studies. Furthermore, due to its shared biological pathways with humans, *C. elegans* serves as an invaluable tool for understanding various fundamental processes including aging and cellular differentiation.
The pharynx itself is a muscular organ that operates through rhythmic pumping movements, leading to food intake. To replicate this mechanism mathematically, Sherman and Harel employed classical mathematics to conduct a bottom-up approach, examining basic configurations such as the various muscle cell types and neuronal signals that control these muscles.
Revolutionizing Biological Experiments
The significance of this modeling approach lies in its ability to conduct complex biological experiments through simulated environments in mere minutes. Traditional laboratory experiments can take years, if they are even feasible, making mathematical simulations a game-changer in fast-tracking our understanding of biological phenomena.
Harel elaborates on this point, explaining that the aim of their model is to mirror the behavior of a naturally-occurring biological system. If the model’s behavior aligns closely with known biological behaviors, researchers can manipulate the system virtually to explore outcomes under varied circumstances—this paves the way for laboratory experiments that can validate or challenge the model's predictions.
A Deeper Dive into Pharyngeal Mechanisms
After six years of dedicated research, the Weizmann Institute’s model provided an extensive analysis of the pharynx, revealing previously uncharacterized dynamics that govern its motion. The findings indicate that the contractions that produce swallowing are not perfectly synchronized; while neural signals broadcast nearly simultaneously, muscle contractions follow at varying paces. This unique model helped clarify these contradictory timings, offering new insights into how these biological systems operate.
Crucially, the model showcased that not only mechanical interactions but also the role of specific ion concentrations is vital for the functioning of pharyngeal muscles, suggesting a broader range of biological interactions than initially understood.
Moreover, the team utilized their model to simulate hypothetical situations, exploring how changes in pharyngeal geometry could impact the worm’s feeding performance—something that would be challenging to test practically in a laboratory.
The Future of Modeling in Biology
With predictions that hold the potential to direct future research endeavors, the impact of *C. elegans* stretches beyond its microscopic size. The insights gained from this research may illuminate the operations of similar biological systems across other organisms, propelling our understanding of life’s intricacies.
By sharing their comprehensive modeling approach, the researchers hope to inspire a wave of interest among nonspecialists in using mathematical models to unlock the mysteries of other biological systems. As curiosity sparks new avenues of research, *C. elegans* continues to prove that sometimes the smallest creatures can shed light on the grandest questions of existence.